Cardiac resynchronisation therapy (CRT) is an established cornerstone of treatment for patients with heart failure (HF) and left ventricular (LV) conduction delay, most typically manifest as left bundle branch block (LBBB). International guidelines make a Class 1A recommendation for CRT device implantation in symptomatic patients with an LV ejection fraction (LVEF) of ≤35%, LBBB and QRS duration (QRSd) of ≥150 ms, despite optimal medical therapy; for the reduction of mortality and morbidity.1,2 The burden of HF remains high even with widespread implementation of CRT in developed countries.3,4
Clinical response rates to CRT have remained largely unchanged since early landmark randomised controlled trials (RCTs) first described their efficacy. Contemporary response rates from clinical trial and real world data demonstrate non-response rates of between 30–50%.5,6
Landmark CRT trials demonstrating reductions in morbidity and mortality all involved some programming optimisation, with or without atrioventricular (AV) delay optimisation using echocardiography Doppler or algorithm-based optimisation methods.7–12 However, no unifying strategy for timing delay optimisation has been included in guidelines due to ongoing debate and conflicting evidence in the literature.
Several common contributory factors have been associated with a lack of response to CRT. Implicated factors include suboptimal AV timing, arrhythmia limiting the percentage of biventricular (BiV) pacing, epicardial LV lead location, suboptimal medical therapy or poor drug compliance, high scar burden and persistent mechanical dyssynchrony. Strategies for improving patient outcomes with CRT and approaches to non-response have been the subject of extensive research.13 Real world data suggest that suboptimal AV timing may be the most prevalent modifiable factor at follow-up.14
CRT programming optimisation is a contentious field due to the increasing complexity of modern devices, pacing algorithm and heterogeneity within the CRT patient cohort. This leads to uncertainty regarding traditional research methodology and the generalisability of RCTs for individualised programming. The physiological ‘sweet spot’ of CRT programming may vary widely for individuals and even across the disease course of the individual.
This review aims to describe advances in programming strategies focused on the utility of the fusion of LV pacing with intrinsic conduction.
Fusion Pacing
Fusion pacing refers to the delivery of CRT pacing with a programming strategy to preserve intrinsic AV conduction via the right bundle branch (RBB). Fusion of the intrinsic activation wavefront with the LV pacing wavefront may be achieved by LV-only pacing or ‘triple fusion’ with the addition of RV pacing. Fusion optimisation methods may have arisen due to a desire to simplify complex echocardiographic optimisation techniques, previously considered to be the gold standard.15–17
Intrinsic Conduction Characteristics
The cardiac excitatory sequence was first mapped ex vivo in eight normal human hearts in 1970 using up to 870 electrodes per heart.18 This work demonstrated that the onset of LV activation is endocardial and trifascicular (anterior para-septal, central left interventricular septal and posterior para-septal) within the first 10 ms. The intricate LV conduction system causes activation to rapidly envelop the majority of the LV cavity within the first 20 ms at a conduction velocity approximating 2 m/s with the posterobasal or posterolateral regions activating last. Activation does not spread across the epicardial surface but from the endocardium to the epicardium.
Adverse remodelling of the failing LV includes progressive dilatation and geometrical changes resulting in eccentric LV hypertrophy, increased wall tension and ultimately myocardial fibrosis. This process contributes to the loss of cardiac output and contractile reserve. The electrophysiological impact is typically manifest as intraventricular conduction delay and the development of bundle branch block due to a disease process affecting the LV conduction system. Unlike the RBB, a relatively delicate structure, the conduction system of the main LBB with its anterior and posterior fascicles, subdividing into the distal Purkinje network is less vulnerable to a focal insult.19 Therefore, a discrete lesion at or just distal to the bundle of His or extensive myocardial insult involving a significant proportion of the conduction system of both fascicles is required for LBBB to manifest. A spectrum of mechanisms and electrocardiographic characteristics are therefore evident in patients diagnosed with LBBB.20
An inverse correlation exists between the magnitude of conduction delay as measured by QRSd and the LV contractile function. Conversely, a positive correlation exists between the prevalence of LV systolic impairment and the presence of LBBB.21 The COMPANION trial showed an incremental increase in the benefit of CRT on mortality and hospitalisation with increasing intrinsic QRSd pre-CRT implantation, a finding supported by the RAFT study.10,22 A meta-analysis of six RCTs and 38 observational studies evaluated the association between baseline and follow-up QRSd with CRT implantation. The RCTs demonstrated that the benefits of CRT appeared restricted to those with a baseline QRSd ≥150 ms. Both broader baseline QRS and a greater magnitude of QRS narrowing were associated with CRT response in the observational studies.23 Additionally, an individual patient data meta-analysis including five RCTs (n=4,317) demonstrated that baseline QRSd was the only predictor by multivariate analysis of the magnitude of CRT effect on outcomes in patients with HF and LV systolic dysfunction in sinus rhythm.24
The MADIT-CRT trial and RAFT study confirmed the importance of QRS morphology and specifically the presence of LBBB to CRT response rates.22,25 Subsequently, varying definitions used for LBBB have been studied and implicated as a key factor in patient selection for CRT. Refined criteria proposed by Strauss et al. for defining complete LBBB as a substrate for CRT, which include sex differences (QRSd ≥140 ms for men and ≥130 ms for women), with mid-QRS notching or slurring in two or more contiguous leads, have been associated with an improved CRT response.26,27
The interval from the onset of the intrinsic QRS on the 12-lead surface ECG to the first large positive or negative peak of the LV electrogram (EGM) (the QLV time) has been investigated as a marker of delayed LV activation and CRT efficacy. QLV times of ≥95 ms have been associated with greater CRT response rates.16,28 With LV lead placement guided by QLV timing of ≥95 ms, the local electrical conduction characteristics have also been shown to be a factor in CRT efficacy. Measurement of the interval from LV pacing stimulation to the QRS onset (S-QRS) <37 ms was associated with increased narrowing of paced QRSd in combination with QLV time >95 ms. This was an independent predictor of CRT response – left ventricular end systolic volume (LVESV) reduction >15% – in a study of 60 HF patients undergoing CRT implantation.29
Despite the value of QLV assessment for LV lead placement, its use when assessing CRT delivery may be limited. A recent retrospective study of 120 patients with HF, LBBB and QRSd >120 ms receiving CRT with quadripolar LV leads describes detailed assessments of intrinsic conduction and ventricular paced effects, measured via device EGMs.30 QLV times were typically similar from distal-proximal quadripolar lead electrodes however correlation was poor between QLV and LV pacing wavefront propagation (LV conduction time) as measured by LVpaced-RVsensed time from device EGMs; regardless of distal versus proximal electrode choice. LV pacing effects varied unpredictably based upon QRS morphology, QLV time and LV stimulation site. They should therefore be considered when individualising CRT programming including when implementing fusion pacing or altering timing (V-V delay) if RV pacing.
QRS Duration as a Target for CRT
Several studies have shown that the magnitude of QRSd narrowing with CRT (intrinsic versus paced) is associated with improved clinical response.31–35 Takenaka et al. demonstrated that QRSd narrowing post CRT was an independent predictor by multivariate analysis of clinical response (LVESV reduction ≥15%).36 The positive association between the magnitude of QRSd narrowing with CRT and clinical response has also been demonstrated retrospectively in patients undergoing upgrade to CRT due to chronic RV pacing.37
The relative change in QRS (QRS index) has also been studied.37,38 A QRS index of ≥10% was significantly associated with CRT response by multivariate analysis in a prospective multicentre study of 311 patients with HF of mixed aetiology.38 Reduction in QRS area as derived from 12-lead ECG by vectorcardiography has also been shown to have a strong association with acute haemodynamic, clinical and echocardiographic response to CRT with additional utility for patients without broad LBBB (<150 ms).39,40
Although single centre studies have disputed the relevance of QRSd narrowing with CRT and patients can respond to CRT without significant QRSd narrowing, accumulating evidence favours this with positive CRT response. Reducing the paced QRSd with device programming adjustment has therefore been investigated as an accessible target for CRT optimisation. The use of fusion of LV or BiV pacing with intrinsic conduction has consistently shown shorter paced QRSd and therefore may be an important strategy for improving CRT delivery.41–44
Fusion Optimised Intervals
Table 1 summarises the key studies assessing fusion optimisation methods. The fusion optimised intervals (FOI) method was first described by Arbelo et al. in 2014, demonstrating greater QRSd narrowing with FOI versus nominal AV delay programming, associated with significant acute improvements in invasive haemodynamics (LV dP/dtmax).41 This method was corroborated in a single centre RCT by Trucco et al. in 2018, showing greater LV reverse remodelling which correlated with QRSd narrowing, by the use of FOI versus nominal programming.42 Ter Horst et al. used a similar methodology testing 20 percentile intervals of the RAsensed-RVsensed time measured from bipolar intracardiac EGMs.45 The cohort’s optimal timing for RV pacing was around the onset of the intrinsic far-field signal (98 ± 17% of RA-RV far-field interval) while preactivating the LV electrode at 50% of the RAsensed-RVsensed interval. VV interval adjustment ranged from 80 ms LV pre-excitation to 40 ms RV pre-excitation in 20 ms increments. The presence of fusion was established by the occurrence of an RV far-field signal prior to the RV pacing artefact during BiV pacing in the absence of a local sensing event. The timing of the far-field signal on RV EGM was verified with that recorded during intrinsic conduction, changes were also confirmed by 12-lead ECG.
These studies used differing approaches to interventricular (VV) interval optimisation and debate persists among studies describing the useful range of VV intervals. Tamborero et al. described 88% (n=25) having optimal VV intervals of <30 ms, but Van Gelder et al. described mean VV intervals of 24 ms ± 33 ms, both optimised by LV dP/dtmax.46,47 Using dP/dtmax to select the optimal VV interval may lead to widely varying values depending on the substrate (ischaemic versus non-ischaemic) and the presence of AF.48 Van Gelder also showed a greater correlation between QRSd and haemodynamic response with QRSd measurement from the first fast deflection of the QRS not the pacing spike.46
The FOI method
FOI involves an ECG assessment of the fusion band: the range of AV intervals in which fusion of intrinsic conduction with LV pacing is present on the 12-lead ECG. The fusion band is established in both atrial sensed and paced modes, starting with the longest AV interval producing consistent LV capture. The AV interval is then sequentially shortened in 20 ms decrements until pure LV capture is identified. The interval with the narrowest QRSd is then chosen as the ‘fusion optimised AV interval’. RV pacing is then introduced and the VV offset altered with LV pre-excitation by 30 ms, simultaneous and RV pre-excitation by 30 ms to find the optimal VV interval. ECG assessments were performed acutely after CRT implantation with QRS measurements at a screen velocity of 300 mm/s although these correlated well with measurements at 50 mm/s. QRSd measurements involved three consecutive cycles, measuring the onset from the start of fast deflection not the pacing spike.
Limitations
Detailed fusion optimisation methods may not fit with many clinical pathways for CRT implantation and optimisation. They involve optimisation of timing intervals at rest, typically supine. The impact of physiological factors including physical activity, and diurnal, postural or vagal variations in heart rate remain largely unknown. Whether the fusion band represents a broad enough interval to allow for these factors and the magnitude of inter and intra-patient variability is also unknown. Patient characteristics for benefit with fusion beyond that of sinus rhythm, intact AV conduction and LBBB are yet to be clearly identified. No unifying definition for intact AV conduction exists within the literature assessing fusion pacing although studies include resting PR intervals of <250–350 ms. This adds some uncertainty to the use of fusion optimisation for patients in sinus rhythm with prolonged PR or right atrium (RA)paced −right ventricle (RV)sensed intervals or those with intermittent AV block. It is also unknown whether patients with non-LBBB intraventricular conduction disturbances benefit from fusion optimisation or indeed whether fusion optimisation methods can be replicated in high degree AV block. Finally, these methods have only been tested in a single centre RCT involving 180 patients.
Mechanism of Fusion Optimisation
It is likely that fusion optimisation directly affects CRT response by several factors. First, fusion optimisation alters the AV delays and, as previously mentioned, suboptimal AV timing is an important driver of reduced response. Second, there is a well-established close correlation between mechanical, contractile ventricular abnormalities and ventricular electrical conduction delay.49 Achieving fusion with intrinsic conduction may contribute to improved mechanics through the correction of electrical ventricular activation patterns, maximising cardiac output, improving LV filling and reducing mitral regurgitation.50,51 Specifically selecting AV intervals with the presence of fusion shortens QRSd, a simplified marker of LV activation time associated with improved CRT response and acute haemodynamics.52
Third, the contribution of the RV contractile function to LV contractility may be reduced by BiV pacing without fusion, conversely fusion of the LV pacing-derived wavefront with intrinsic RBB conduction may be required for maximum acute response.53,54 This is supported by an invasive electro-anatomical contact mapping study which demonstrated an association between the presence of fusion of intrinsic activation following echo-guided AV optimisation and a higher rate of LV and RV systolic function improvement at 6 months (n=8).55 Therefore the presence of intact conduction over the RBB leads to rapid RV activation and partial LV activation depending on the level and extent of conduction block present in LBBB.45 The potential benefits of fusion optimisation and mechanism of efficacy in selected patients are not fully explained and therefore require further study.
Electrogram Algorithm-based Programming Optimisation
Static Electrogram Algorithms
Table 2 summarises algorithms aiming to target fusion which have been studied and largely implemented into routine device care. Preliminary studies often employed a static programming approach with optimisation techniques including infrequent or ‘one-off’ adjustments. These algorithms share some limitations with invasive haemodynamic and echocardiographic optimisation studies.
The SMART-AV trial randomised 980 patients 1:1:1 to the SmartDelay electrogram AV optimisation algorithm (Boston Scientific) versus echocardiographic AV optimisation versus nominal AV interval programming (120 ms). At 6 months there were no differences in the primary endpoint of LV reverse remodelling or secondary endpoints of clinical response, including New York Heart Association (NYHA) class, 6-minute walk distance and quality of life score.16 This juxtaposed the positive outcomes from an acute optimisation study comparing echo versus dP/dtmax versus SmartDelay AV interval optimisation in 28 patients. The results showed that the algorithm accurately predicted both echocardiographic and invasive haemodynamically optimal AV intervals.16,56
Sub-analysis of the SMART-AV trial has been performed comparing patients with nominal AV intervals versus SmartDelay optimised intervals.57 This study found that reverse remodelling with CRT, as assessed by changes in LVESV >15%, was strongly associated with the sensed RV-LV interval duration (the time between the peaks of the RV and LV EGMs) and that CRT response increased with RV-LV prolongation for both programming sub-groups. Patients with longer RV-LV intervals had greater benefit from AV interval optimisation versus nominal programming. Patients with the longest RV-LV durations (fourth quartile, ≥105ms) had 4.26 times greater odds of an LVESV response with AV optimisation versus nominal programming (p=0.01).
Dynamic Electrogram Algorithms
Figure 1 displays a practical example of the use of a dynamic AV delay algorithm targeting fusion to achieve maximal QRS narrowing, in this case with an offset of -10 ms from the intrinsic AV delay. Table 2 summarises the available dynamic algorithms for managing AV interval timing in CRT. The AdaptivCRT (aCRT) algorithm was evaluated in a well-constructed prospective, multicentre, double-blind RCT.58 Subgroups with the greatest treatment effect were those with prolonged intrinsic AV intervals and significant reverse remodelling of the left atrium. Large retrospective registry datasets subsequently support these outcomes showing an association between aCRT, improved survival and reduced burden of AF.59,60 Recent analysis of this study shows that non-physiological AV programming is associated with an increased incidence of AF.61 The AdaptResponse trial (NCT02205359), a multicentre RCT has completed enrolment of 3,620 CRT-indicated patients with symptomatic HF, NYHA II–IV, LBBB (QRSd ≥140 ms in men; ≥130 ms in women, according to Strauss criteria) and PR interval ≤200 ms in sinus rhythm.62 This trial randomised patients 1:1 to aCRT on versus off (standard BiV CRT) and will report on a combined primary endpoint of all-cause mortality and intervention for decompensated HF at 2 years post-randomisation in late 2023 or early 2024.
The SyncAV algorithm’s function is illustrated in Figure 2. Its use has been associated with improvements in electrical synchrony as measured by QRSd in patients with LBBB in sinus rhythm.43,44,63 These studies consistently demonstrated the potential for additional incremental QRSd narrowing achieved in patients with tailored SyncAV offsets during BiV pacing. Recently published data by O’Donnell et al. showed the effect may be further augmented with the addition of multipoint pacing (MPP).64 The impact of SyncAV programming on acute haemodynamic response has also been assessed non-invasively using aortic velocity time integral (VTI) and systolic blood pressure response, with augmentation of response seen using personalised SyncAV offsets.65,66
Hard outcome data from a well-designed RCT assessing the impact of SyncAV in CRT is awaited. The LV-only MPP with SyncAV study (NCT03567096) is currently recruiting patients with de novo CRT implants in sinus rhythm with LBBB randomised 1:1 to biventricular MPP or LV-only MPP using simultaneous V-V delays and widest anatomical LV electrode separation (≥30 mm). Response will be assessed by clinical composite score (CCS) at 6 months following optimisation of SyncAV offset targeting the narrowest QRSd. This study will give further insights into the use of this dynamic device-based algorithm to achieve fusion pacing in the context of MPP. Additionally, the SyncAV post-market trial is a multicentre, open-label RCT actively recruiting to a target of 1,300 patients with symptomatic HF, LBBB (QRSd >120 ms) and intact AV conduction (PR interval <280 ms). Randomisation by 1:1 assignment to SyncAV on versus off (standard BiV fixed AV delays) with SyncAV offsets programmed to produce maximal QRSd narrowing. Optimisation will include assessment of LV only, RV ahead 30 ms and LV ahead 30 ms pacing; with repeat SyncAV optimisation at 3 and 6 months. The primary outcome measured will involve change in LVESV at 12 months assessed by echocardiography.

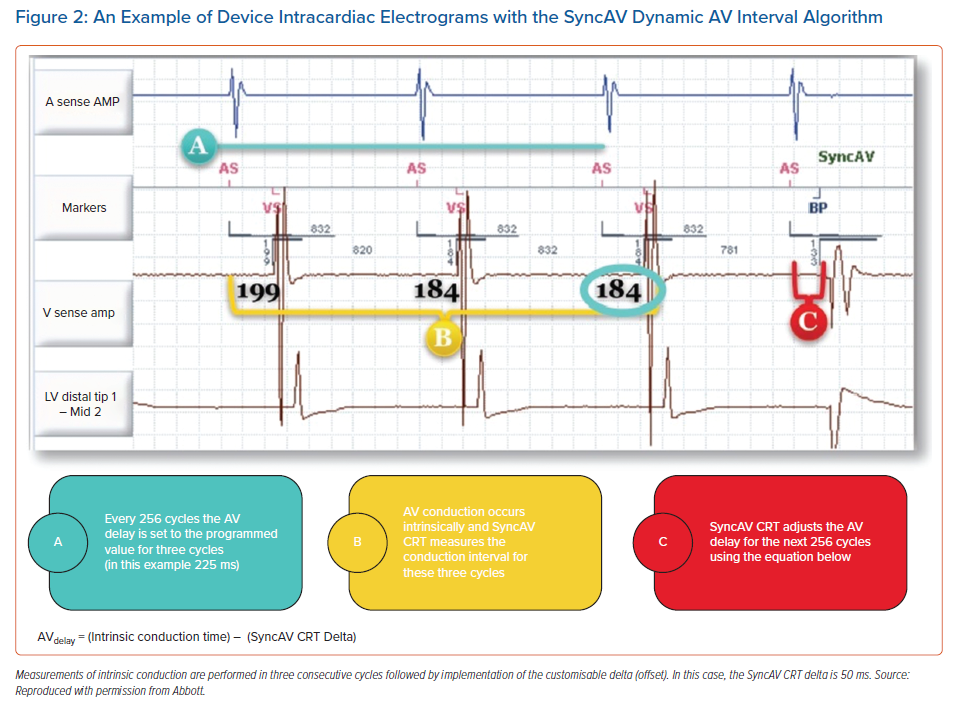
The Role of Mapping in CRT
Invasive Mapping
In vivo mapping of LBBB in 24 patients with HF using invasive contact and non-contact mapping was published by Auricchio et al. in 2004.67 This work demonstrated a U-shaped LV activation pattern due to a line of functional transmural conduction block. The location of conduction block was variable but present between the LV septum and lateral wall. Lambiase et al. performed the first invasive non-contact endocardial mapping study investigating the importance of LV lead position in 10 patients with recent CRT device implantation.68 The results demonstrated that endocardial pacing in regions of slow conduction produced delayed progression of the depolarisation wavefront. This affected the efficacy of resynchronisation of LV activation, resulting in persistence of dyssynchrony and lack of positive acute haemodynamic response. Thus, avoidance of regions of slow conduction through mapping significantly improved the acute haemodynamic response to CRT. This study also suggested that LV activation time is a more precise marker of resynchronisation than QRSd. Knowledge of the pattern of LV activation, location of conduction block and whether it is fixed or functional in response to LV pacing may allow better understanding of a poor response to CRT.
Non-invasive Mapping: Electrocardiographic Imaging in CRT
Electrocardiographic imaging (ECGi) is a non-invasive solution to invasive cardiac mapping, avoiding risks of arterial puncture and cardiac catheterisation. A vest applied to the torso with multiple electrodes (ranging from 50–300 depending on the manufacturer) records body surface EGMs via a specialised mapping system. Cross-sectional imaging using CT or MRI with the electrodes in situ acquires the 3D cardiac torso geometry necessary to compute epicardial potentials from body surface potentials using a mathematical inverse solution.69,70 This provides whole-heart imaging superior to conventional electrocardiography, allowing identification and quantification of global ventricular activation patterns and local events with high resolution (≤10 mm for earliest and latest sites), validated in vitro and in vivo.71, 72
Non-invasive ECGi mapping has demonstrated heterogenous activation patterns among patients with HF and BBB, replicating invasive studies.73 ECGi has been used both to refine prediction of CRT response and as part of a multi-modality imaging assessment at the time of device implant to aid LV lead delivery.74
The impact of ECGi-guided programming optimisation for conventional BiV pacing, His-Bundle pacing and MPP is currently being evaluated.75–77 ECGi using the CardioInsight system (Medtronic) has suggested a reduction in global LV activation times through the use of MPP versus BiV pacing.76 ECGi has not yet been used to assess the impact or mechanism of fusion optimisation. Pre-implantation prediction of response to CRT using ventricular electrical uncoupling time (the difference between mean LV and RV activation times) >50 ms, achieved a 90% sensitivity and 82% specificity for predicting CRT response in 33 patients with mixed intra-ventricular conduction delay and QRSd >120 ms.78
Potential applications for ECGi involve pre-implantation prediction of response and procedural planning including guidance of LV lead delivery to LV segments of latest electrical activation away from zones of slow conduction. Post-implant mapping of the LV pacing wave front propagation (‘paced effects’) outside the catheter laboratory may provide valuable data to deliver individualised CRT programming including fusion optimisation as well as investigating non-response.
LV-only Pacing
LV-only pacing to fuse with the intrinsic activation (LVp), produces a double wave front of ventricular activation. This may avoid potentially adverse RV pacing-induced dyssynchrony. LVp is non-inferior to BiVp in patients with QRSd ≥120 ms and conventional indications for CRT in terms of exercise capacity, peak oxygen consumption, NYHA class, LVEF and acute haemodynamics measured invasively.79–84 The evidence for non-inferiority in patients with intact AV conduction and broad QRS includes high quality double blinded RCTs and a meta-analysis, leading to its use being endorsed by the European Society of Cardiology in CRT guidelines.84,85
Long-term clinical implications of LV-only pacing have been tested in the multi-centre double-blinded crossover GREATER-EARTH trial involving 211 patients with de novo CRT implantation randomised 1:1 to LV and BiV pacing for consecutive periods with 6 months crossover.79 The results showed non-inferiority of the primary outcome of exercise capacity and secondary outcome of reverse LV remodelling. After crossover >20% of the BiV pacing group became responders with LVp suggesting a role for LVp in non-responders to BiV pacing as an alternative therapy. A critique of this study design is that the AV delays were optimised to avoid fusion by programming AV intervals with complete LV capture and mean AV intervals of 101 ± 16 ms.
LV univentricular/mono-ventricular pacing is a strategy involving the implant of a dual chamber pacemaker or CRT device with an LV epicardial lead and no RV lead. This is an option occasionally implemented in patients without HF but with anatomical constraints such as significant tricuspid valve pathology/replacement or congenital heart disease. It has also been explored for HF patients with CRT indications in developing countries with restricted resources where dual chamber pacemakers have been implanted with LV leads for patients with HF, LBBB and intact AV conduction.86 This non-randomised study included 30 patients receiving LV univentricular pacemakers and showed non-inferiority by clinical and echo criteria but significant cost savings at 6 months versus standard BiV pacing with fixed AV intervals. AV delay optimisation was performed using echo and 10 ms decrements from the resting PR interval prior to initiating rate adaptive AV delay algorithms.
Although this option exists, widespread implementation is likely to remain limited due to the lack of RV lead required to sense ventricular events, defibrillate and provide backup pacing if the LV lead fails.
Multipoint Pacing
MPP refers to CRT involving epicardial stimulation from more than one pole of a multipolar (typically quadripolar) LV lead. O’Donnell et al. have shown the incremental benefit to QRSd reduction of MPP versus BiV pacing when used in combination with SyncAV in patients with LBBB and PR interval of ≤300 ms. Several studies have demonstrated improvements in acute haemodynamic, echocardiographic, clinical response and QRSd reduction with MPP versus BiV however the true efficacy of MPP remains uncertain due a lack of prospective multicentre RCT trial data showing treatment benefit.87
A systematic review and meta-analysis of 11 studies assessing MPP versus conventional BiV pacing demonstrated reduced HF hospitalisations, improved LVEF, improved CRT clinical response, decreased all-cause morbidity and cardiovascular mortality by sub-group analyses. This included variable study sizes and designs, limiting the applicability of this meta-analysis.88 Programming strategies varied across all included studies with regards to AV, VV intervals and LV pacing vectors, further limiting any conclusion regarding fusion pacing strategies with MPP.
Any incremental benefits from MPP are likely to depend on factors including LV lead location, proximity to myocardial scar or regions of slow conduction, LV lead pacing thresholds, presence of phrenic nerve stimulation as well as appropriate AV and VV timing delay programming. Another study has suggested that AV intervals with optimal invasive haemodynamic response are similar in all electrodes of a quadripolar LV lead and that AV optimisation may only need to be performed in one electrode including for the use of MPP.89 This study indirectly assessed the impact of fusion with intrinsic conduction by 20% increments of the RApaced-RVsensed interval during AV optimisation and concluded that 50% of this interval was correlated with optimal haemodynamics by stroke work; assessment of change in QRSd or presence of fusion was not included.
The use of MPP has been studied specifically for CRT non-responders in the MORE-CRT MPP study.90 Non-responders (<15% reduction in LVESV) were randomised 1:1 at 6 months follow-up post CRT implant to receive either MPP or continued conventional BiV pacing. MPP did not significantly increase the proportion of echocardiographic responders following a further 6-month period. Programming strategies were left to the physician’s discretion, however sub-group analysis showed that MPP programmed with a wide LV electrode anatomical separation (≥ 30 mm, MPP-AS) and shortest interventricular timing delays appeared to have the greatest incremental benefit in clinical response compared to other MPP programming (45.6% versus 26.2%, p=0.006).
The MPP IDE trial was a prospective, double-blinded, multicentre RCT with 469 participants using a complex design to assess MPP as a therapy for non-responders to CRT, defined at 3 months post-implant by CCS.91 After 3 months of BiV pacing, patients underwent echo assessment including Doppler of the transmitral flow (EA VTI) and only those with EA VTI during MPP ³BiV pacing were randomised 1:1 to receive continued BiV pacing versus MPP from 3–9 months. MPP programming was left to the physician’s discretion. The primary endpoint of non-inferiority and freedom from system-related complication was met with no significant difference between the responder rates of MPP and BiV pacing at 9 months. The incidence of incremental CRT response was, however, significantly higher in patients with MPP using the widest anatomical separation (≥30 mm) of LV electrodes (MPP-AS) at shortest interventricular delay (5 ms) versus MPP-other, 54% (28/52) versus 41% (61/147) (p=0.008).
Varma et al. have recently published further analysis of the MPP IDE study with patients dichotomised by median baseline left ventricular end diastolic volume index (LVEDVI) to height (LVEDVImedian).92 Patients with a higher than median LVEDVI had lower response rates to BiV at 3 months by CCS (65% versus 79%). MPP-AS programming produced greater CCS response rates versus BiV at 9 months (92% versus 65%, p=0.023). With LVEDV>Median, HF event rates increased between 3–9 months with BiV but stabilised with MPP-AS. This study concluded that patients programmed to MPP-AS after 3 months of BiV had a greater response and those with larger hearts (by LVEDVI) had lower response rates with BiV via a quadripolar lead.
The study is limited by its complex design and the selection criteria used for randomisation (positive EA VTI response to MPP) perhaps selecting patients more likely to respond. The study did not mandate programming for MPP therefore it is unclear whether AV intervals were programmed with any specific goal such as fusion.
Given the growing data suggesting benefit with MPP (specifically MPP-AS), the combination of fusion with intrinsic conduction and dual-site LV stimulation may achieve the most comprehensive electrical resynchronisation for selected patients. This strategy requires testing in a well-constructed prospective, randomised study.
Emerging Strategies and Future Directions
Although fusion optimisation of CRT using epicardial LV leads may provide additional benefit for patient outcomes, limitations remain in a significant minority of patients due to the epicardial location of the LV-pacing electrode. Several emerging strategies have sought to overcome these limitations through direct stimulation of the proximal conduction system with His-Bundle or LBB pacing and the distal conduction system with endocardial pacing. Physiological phenotyping by invasive electrophysiological testing has also shown potential by characterisation of the underlying pathophysiology. This may allow targeted and more complete electrical resynchronisation through conduction system (His bundle/LBB) pacing in selected patients.93 Additionally, non-invasive mapping (ECGi) is a powerful tool with the potential to improve assessment of non-responders and patient selection for CRT.
With the emergence of artificial intelligence and machine learning into healthcare, advanced CRT devices could use feedback and learning loops to maintain pre-specified goals such as minimum QRSd and desired QRS morphology from continuous EGM analysis. Dynamic offsets could be applied with the ability to maintain fusion throughout the individual’s heart rate range.
Conclusion
Despite advances in CRT device technology and improved understanding of the complexity of HF with conduction delay, response rates and longevity of response remain relatively unchanged. This review specifically addresses the concept of fusion optimisation as a method for improving patient outcomes with CRT. LV-only pacing is an established alternative to BiV pacing and may be considered in a tiered fashion for BiV non-responders with fusion optimisation maximising individual response. The addition of MPP-AS to fusion may produce the greatest electrical synchrony, however, definitive long-term outcome data are awaited and a well-constructed prospective RCT assessing clinical outcomes with this strategy would be of value.
Dynamic algorithm-based optimisation targeting narrowest QRSd for patients in sinus rhythm with intact AV conduction and LBBB shows promise as a strategy for improving CRT delivery. The aCRT algorithm has been shown to be clinically beneficial, particularly when RV-LV conduction times are prolonged. The SyncAV algorithm appears to incrementally improve electrical synchrony; however clinical outcome data are awaited. Both avoid the need for detailed electrocardiographic or invasive assessment. Gaps in the literature remain regarding the use of fusion optimisation in non-LBBB conduction delay, AV block, chronic AF and during MPP.
Clinical Perspective
- Suboptimal atrioventricular timing may be the most prevalent modifiable factor influencing clinical response to cardiac resynchronisation therapy (CRT).
- The magnitude of the QRS duration reduction with CRT is associated with improved outcomes.
- Greater QRS narrowing may be achieved by fusion of left ventricular pacing with intrinsic conduction.
- Fusion pacing appears to be effective in patients with intact atrioventricular conduction and broad left bundle branch block. The effect may be augmented by the addition of multipoint pacing.