Mitral valve prolapse (MVP) is the most common valvular heart disease and is estimated to affect 2–3% of the general population.1 Barlow’s disease is a clinical syndrome characterised by MVP, a late systolic murmur and nonejection systolic click; the cornerstone of the disease is a fibro-myxomatous modification in the mitral leaflet tissue, with superior displacement of one or both leaflets into the left atrium.2,3 Although MVP has generally been regarded as a benign condition, several studies have reported ventricular arrhythmias (VAs) and sudden cardiac death (SCD).4–7 Basso et al. detected left ventricular (LV) fibrosis at post mortem examination at the level of the papillary muscle (PM) and/or infero-basal wall.8 LV late gadolinium enhancement (LGE) was identified by contrast-enhanced cardiac magnetic resonance (CMR) in the same regions.
The aim of this article is to summarise the pathophysiology, risk stratification and available treatment options for patients with MVP and VAs.
Arrhythmogenesis and Electrophysiological Substrate
Arrhythmogenesis in MVP is the result of a complex interplay between the substrate and a mechanical and electrical trigger, which goes beyond the PM anatomy. The most common arrhythmic presentation in MVP is premature ventricular complexes (PVCs), followed by non-sustained ventricular tachycardia (VT), sustained VT, polymorphic VT and VF.
Several animal models of mechanical stretch-induced arrhythmias have been proposed. These models might also provide some hints on the pathogenesis of MVP-related arrhythmias. In a canine model, Gornick et al. demonstrated that the mechanical traction of PM was associated with local premature ventricular activation.9 Other studies in cardiac tissues showed that myocardial stretch is associated with reversible depolarisation of transmembrane potential, with the latter being associated with both transient afterpotentials and propagated action potentials.10,11 Stretch-induced depolarisation may induce PVCs that can be the main clinical finding or the trigger for malignant arrhythmias.12,13 Furthermore, a prolongation of the ventricular functional refractory period has been demonstrated in the traction zone. Regional refractory period prolongation may cause action potential heterogeneities, demonstrated to serve both as the substrate for polymorphic VT and as the trigger for short-coupled PVCs (‘R from T mechanism’).14 In a study based on 2D and 3D simulations, large heterogeneities were implicated in the initiation of focal activity polymorphic VT and smaller heterogeneities in re-entry-type polymorphic VT.15 The final result of those action potential heterogeneities was polymorphic VTs induced both by multiple competing foci and re-entry circuits.
Beyond the PM structure, the Purkinje system (PS) may be implicated as a substrate and a trigger of malignant MVP. Distal arborisations of PS are localised near the base of the PM, where Purkinje-like potentials can often be recorded.16 PS-ventricular muscle junction is a region characterised by a low safety factor for propagation. The safety factor is defined as the ratio between the upstream current and the downstream threshold current.17 A safety factor of at least 1 is necessary for conduction.
A low safety factor in PS-ventricular muscle junction can be explained by the narrow curvature of the propagation wavefront in this region.18 This can lead to ‘source-sink mismatch’, propagation failure and predisposes to reentrant arrhythmias. In a 3D model of polymorphic VT, reentrant excitation was initiated in the myocardium of the LV by unidirectional propagation; the PS was demonstrated to be necessary for the maintenance of that reentry, at least in the initial stage.19 Furthermore, a ‘source-sink mismatch’ was also demonstrated in the PM region, secondary to an abrupt change of the muscular fibre orientation and also related to the electrical mass of PM, which serves as an additional sink. 20 Based on these observations, Kim et al. demonstrated in a swine model that during VF, reentrant wavefronts are often transiently anchored to the PM. Purkinje fibre potentials were recorded at the base of PM and preceded the local myocardial activation. Tissue mass reduction converted VF to VT, while PM disconnection led to the non-inducibility of sustained VT.21
In a series by Santoro et al., the main mechanism of VF originating from the PM was short coupled PVCs that could be preceded by Purkinje-like potentials.22 However, Purkinje-like potentials precede local ventricular activation during sinus rhythm but are bystander activated during PVCs, suggesting that PVCs originate from the ventricular PM, rather than from the conduction system.23 Thus, based on anatomical proximity, PS might be implicated in enabling and maintenance of reentrant mechanism initiated by PM.
Since the first description of arrhythmic MVP, a substrate could be demonstrated based on histopathology and CMR. In their seminal study on SCD in MVP, Basso et al. detected endo-perimysial and patchy replacement-type fibrosis at the level of PM in all SCD patients, and sub-endocardial/mid-mural fibrosis in the infero-basal LV wall under the posterior mitral valve leaflet in 88% of cases. 8 In vivo CMR demonstrated LV LGE in 93% of arrhythmic MVP versus 14% of control subjects, with a regional distribution overlapping with the histopathological findings. Furthermore, in a large primary mitral regurgitation cohort, CMR LGE appeared as an important prognostic factor, with the highest arrhythmic event rate seen in MVP patients with replacement fibrosis (7.7%), followed by MVP patients without replacement fibrosis (2.7%) and non-MVP patients (0.6%).24
When considering programmed electrical stimulation and invasive electroanatomic mapping, Syed et al. found that bipolar and unipolar voltage maps were normal in all MVP patients undergoing VAs ablation; abnormal LV fascicular potentials, defined as fractionated, delayed, or mid-diastolic Purkinje electrograms, were evident in all patients with MVP and previous cardiac arrest, and in all cases of sustained VAs induced during the electrophysiological study.25
Risk Stratification
MVP is an under-recognised cause of SCD in young adults, accounting for 7% of total events and 13% in females.8 Several small cohort studies have attempted to identify patient characteristics associated with major cardiac events. However, to date, results are contradictory and a comprehensive risk stratification is not available. We will review the results of the most important studies on the topic. Risk factors are summarised in Figure 1. Avierinos et al. suggested that female sex and young age are associated with an increased arrhythmic risk.26 Bileaflet MVP was frequently found in patients experiencing idiopathic out of hospital cardiac arrest (in the absence of ischaemia, cardiomyopathy and channelopathies). Other common characteristics in this subset of patients were the presence of isodiphasic or negative T waves in inferior leads, PVCs with outflow tract, PM or fascicular origin.27 At ECG evaluation, the presence of T wave abnormalities in the inferior leads (inverted or isodiphasic T waves) was found in >80% of the cases of MVP-related SCD.8 Wei et al. showed that a large burden of PVCs was associated with higher rate of non-sustained and sustained VT occurrence.28 Complex arrhythmias, such as pleomorphic PVCs with alternating morphology, ventricular couplets or triplets, are much more frequent in the MVP population than in the general population.29 They are a possible trigger for malignant arrhythmias.
In a large cohort of 595 consecutive patients, 9% had severe VAs (VT ≥180 BPM and/or history of proven VT/VF), 27% had moderate VAs (VT 120–179 BPM), 8% had mild VAs (≥5% PVC and/or VT <120 BPM), and 57% had no/trivial ectopy (<5% PVCs). Arrhythmia severity was strongly associated with adverse outcome, with mortality rates at 8 years after the diagnosis ranging from 10 ± 2% in patients with no/trivial VAs, to 15 ± 3% for mild and/or moderate and 24 ± 7% for severe arrhythmia (p=0.02). Severe VA was independently associated with mortality after adjustment for clinical covariates (adjusted HR 2.94; 95% CI [1.36–6.36]; p=0.006).30
Cardiac Imaging Characteristics
Cardiac imaging with trans-thoracic, trans-oesophageal echocardiography and CMR provides valuable support for correct risk stratification. As early as 1994, Zuppiroli et al. demonstrated the relationship between mitral valve tissue redundancy – in particular anterior mitral leaflet thickening – with the occurrence of complex VAs.31 Moreover, MVP-related regurgitation appeared as an independent predictor of VAs.29 Contradictory studies are available regarding the role of surgical treatment of mitral regurgitation in prevention of major arrhythmic events. Naksuk et al. showed that surgery did not uniformly reduce the PVC burden, but the reduction was greater in young patients, thus suggesting a possible benefit on arrhythmic burden from early surgical referral.27
The presence of mitral annular disjunction (MAD), defined as the detachment of the mitral annulus compared to the ventricular myocardium during cardiac systole, and the presence of the Pickelhaube sign, defined as an high-velocity positive systolic wave at tissue Doppler evaluation of lateral mitral annulus, also characterised a cohort of patients with a high risk of malignant VAs.32–34 The clinical role of MAD is not yet fully understood. In particular, Dejgaard et al. showed that MAD was associated with VAs, even in absence of detectable MVP, thus suggesting that MAD itself is an arrhythmogenic entity.34 Arrhythmia risk increased proportionally to MAD length, suggesting a central role of MAD in the arrhythmogenic MVP.35 However, it is also possible that MAD and overt MVP are components of the same clinical spectrum.36 In 2017, Muthukumar et al. described the Pickelhaube sign. During mid-systole, the excessive movement of the prolapsing mitral valve causes traction on the myocardium of the inferior-lateral wall and on the PM; this mechanical event can cause a local electrical dysfunction, even in the absence of fibrosis detected by CMR.25,33
CMR has shown an additive role to echocardiography in the risk stratification of patients with MVP. Basso et al., by histological evaluation, detected the presence of fibrosis at the level of PM and inferior-basal wall in most patients with MVP. 37 In the same cohort of patients, the presence of LGE was associated with complex VA occurrence; LGE distribution was similar to the one found by histopathological examination.37 In patients with primary mitral regurgitation, LV myocardial fibrosis is more frequent in MVP than in non-MVP patients; myocardial fibrosis appeared as an independent arrhythmic risk factor.24 This correlation was also reported by Han et al., who identified focal regions of LGE in the PM in 63% of their cohort; this finding was associated with the presence of complex VAs.36 Arrhythmic events in MVP patients were also associated with the presence of MAD detected by CMR, larger end-systolic and end-diastolic mitral annular diameters, posterior systolic curling and basal to mid LV wall thickness ratio >1.5.37 Among patients with MAD, Scheirlynck et al. found VAs in 22 (31%). The implementation of additional risk factors allowed a more appropriate stratification of patients with MAD. The risk of VAs was highest in those with LGE at the PM, high levels of soluble suppression of tumourigenicity-2 and reduced LV ejection fraction (LVEF).38 The excessive movement and mechanical stretching present in the pathological mitral valve systems favour LV hypertrophy, myocardial scarring and, consequently, the occurrence of VAs.
To date there is no shared expert consensus on the management of patients with MVP and risk of VAs. Because several grey areas still exist in risk stratification and no diagnostic methodology has been extensively validated, a multimodal evaluation becomes fundamental. Our current diagnostic practice includes the use of periodical ambulatory ECG monitoring or implantable loop recorders in MVP patients symptomatic for palpitation, in the absence of any risk factors. We tend to perform CMR before programmed electrical stimulation in patients with complex PVCs or syncope. In patients with aborted SCD, an ICD is mandatory. Catheter ablation of the documented VAs is a promising therapeutic option. However, any potential beneficial effects on patient survival have not yet been clearly documented.
Treatment
Although the arrhythmogenic role of PM has been well known since the 1980s, catheter ablation of VAs originating from PM is a relatively recent acquisition. First experiences, dating to the late 2000s, reported PM-related VAs both in the context of ischaemic cardiomyopathy and in normal hearts, with a spectrum of clinical presentation ranging from isolated PVCs to non-sustained VT, sustained VT and VF.22,39,40
Doppalapudi et al. described a cohort of seven patients out of 290 referred for the ablation of idiopathic VAs.23 Patients showed the following distinctive characteristics, which suggested the presence of a new clinical syndrome: normal baseline ECG and intracardiac conduction intervals, with normal LV systolic function; right bundle branch block and superior-axis PVC morphology; lack of VA inducibility by electrophysiological study and atrial programmed electrical stimulation; VT or PVC inducibility by intravenous isoproterenol or epinephrine; earliest ventricular activation at the base of the posterior PM in the LV; and absence of high-frequency potentials at the site of origin, thus suggesting that the PS was not directly involved. VAs originating from the anterior PM were later described by the same group.41
Among a cohort of 597 patients treated for VAs, Enriquez et al. identified 25 patients with MVP and PVCs mapped to the PM.42 The clinical presentation was PVC-triggered VF in four of them and one patient died during follow-up. CMR and electroanatomic maps were available in a small subset of cases (nine and 11 patients, respectively). Bipolar voltage abnormalities were identified only in three patients. Acute ablation procedure was associated with a significant reduction in PVC burden during follow-up (from 20.4% ± 10.8% to 6.3% ± 9.5%; p=0.001) and with improvement of post-ablation LV function in patients with depressed LVEF (five of six patients).
ECG Criteria for the Identification of the Exit Point of Premature Ventricular Complexes
When planning catheter ablation in patients with MVP, analysis of ECG morphology might help differentiating PM VAs from other arrhythmias presenting with right-bundle block morphology, i.e. LV fascicular and mitral annulus related arrhythmias.41,43,44 Typically, VAs originating from the posteromedial PM, posterior fascicle or the posterior mitral annulus exhibit a superior axis, while those originating from the anterolateral PM, anterior fascicle or the anterior part of the MA, present with an inferior axis. QRS is significantly wider in patients with PM arrhythmias compared with the fascicular ones. All fascicular arrhythmias share an rsR’ morphology pattern in lead V1, which is not present in the arrhythmias originating from the PM.45 In order to discriminate PM and LV fascicular from mitral annulus VAs, Yamada et al. proposed several ECG criteria.46 Among them, only an R/S ratio ≤1 in lead V6 in the LV anterolateral region and a QRS duration >160 ms in the LV postero-septal region were reliable predictors of PM exit. More recently, Al’Aref proposed an ECG algorithm based on QRS duration, r and R’ amplitudes in V1 and precordial transition to differentiate the three subgroups.44
When clinically applying the previously cited ECG criteria, the electrophysiologist should be aware that they present some potential limitations. In fact, the criteria have not been validated in specific populations with confirmed diagnosis of MVP. Theoretically, PMs VA should share a common exit located at the base of the PM. However, several anatomical configurations have been described. PM portions might be connected with other portions of the same PM, with the opposite PM, with the ipsilateral or contralateral LV wall and bifid PMs might present a mismatch between the strands. Rivera et al. found PM connections in 62% of PMs from patients undergoing VA ablation. 47 The PM connections might be responsible for impulse propagation in areas located away from the PM base, thus providing an R/S transition in the precordial leads and/or a QRS axis inconsistent with the site of VA origin.
Electroanatomical Mapping and Catheter Ablation
Mapping and ablation of VA in MVP are usually performed with standard electrophysiological techniques. Nevertheless, the particular location of the substrate requires the implementation of specific arrangements. Idiopathic PM-related VAs usually exhibit a focal, non-reentrant mechanism (automaticity or triggered activity); ventricular/atrial programmed electrical stimulation are usually not able to induce those arrhythmias. Electroanatomic mapping of VAs originating from PM is affected by several issues, including the presence of a deep focus within the PM and possibly multiple exit points. In almost half of the patients treated by Yamada et al., PM VAs exhibited multiple QRS morphologies, often requiring radiofrequency lesions on both sides of the PM in order to completely eliminate the VAs.40 Catheter ablation guided by pace-mapping is therefore hampered by a high risk of failure. Activation mapping is the most commonly used mapping approach: the aim is to target the earliest pre-potential bipolar activity (≥30 ms before the QRS onset) or Purkinje-like potentials, which can be found in about 40% of patients (Figure 2).46 Isoproterenol or epinephrine infusion and burst pacing from right ventricular apex/right atrium can be eventually useful to increase PVCs frequency. Enriquez et al. mapped 27 different PM PVCs in 25 patients.42 The site of PVC origin was the posteromedial PM in 14 patients (56%), the anterolateral PM in eight (32%) and both PMs in three patients (12%). In sinus rhythm, a Purkinje potential was recorded at the site of successful ablation in seven cases; in the other two patients a late potential was recorded in sinus rhythm that became pre-systolic during the PVC.42
After the identification of the arrhythmia exit point, the ablation phase is also hampered by a series of technical difficulties. The arrhythmic focus is usually localised but deep in the myocardium. The PM is an anatomically complex 3D structure, protruding from the myocardial wall into the LV cavity, changing its position at every heartbeat. It is also in close proximity with other structures of the mitral apparatus (mitral valve chordae and leaflets), which might be damaged by radiofrequency. A trans-septal approach with steerable sheets can improve catheter stability and is usually advisable. Intracardiac echocardiography allows the direct visualisation of the PM during mapping and helps to correctly identify the anatomical localisation of the arrhythmogenic focus; during catheter ablation, it allows confirmation of contact between the ablation catheter and the target PM, avoiding damage to surrounding anatomical structures.23,48
The complexity of the substrate in VAs arising from PM justifies the low acute success and high recurrence rate after catheter ablation. In a large multicentre analysis of idiopathic PVCs ablation procedures, PM-related VAs showed the second lowest acute procedural success (80%), after epicardial foci (67%). During follow-up, patients with PM-related PVCs had among the highest recurrence rate, with only 60% of patients maintaining ≥80% reduction in PVC burden without antiarrhythmic drugs.49 Small cohort studies have evaluated the predictors of successful ablation; these included the smaller size of the PMs assessed by CMR, and the presence of Purkinje potentials at the site of ablation, which may reflect a superficial location of the arrhythmogenic substrate.49
Cryoablation, theoretically, provides the advantage over radiofrequency catheter ablation of greater catheter stability, deeper and more homogeneous lesions. However, in the clinical setting conflicting results have been reported concerning any real benefits. In a small series by Rivera et al., the acute success rate was 100% for cryoenergy in 12 patients and 78% for radiofrequency in nine patients (p=0.08).50 The better acute results of cryoenergy were probably related to a higher catheter stability achieved with cryoenergy (100% of treated patients), than radiofrequency (25% of treated patients, p=0.001). VA recurrence at 6-month follow-up was significantly lower in patients treated with cryoablation (0%), than those treated with radiofrequency (44%; p=0.03). Widespread application of contact-force monitoring for catheter ablation procedures significantly improved procedural endpoints and, in cases of PM-related arrhythmias, it contributed to bridge the gap with cryoenergy. The same authors compared three techniques for ablation of PM-related arrhythmia.47 These were cryoablation with cardiac CT integration into the electroanatomical mapping system, radiofrequency ablation with non-contact-force-sensing catheters and cardiac CT integration, and contact-force-sensing radiofrequency ablation catheters with intracardiac echo-facilitated 3D electroanatomical mapping. Acute success and arrhythmia recurrence during follow-up were 100% (14 patients) and 7% (one patient), respectively, with contact-force-sensing radiofrequency ablation catheters/intracardiac echo. Cryoablation/cardiac CT achieved non-significantly different results with acute success in 100% (16 patients) and recurrence rates in 19% (three patients). Non-contact-force-sensing radiofrequency ablation was associated with a reduced acute success rate (19 patients; 83%; p=0.03) and an increased risk of clinical arrhythmia recurrence (11 patients; 48%; p=0.02). Cryoablation has also been proposed in patients with arrhythmia recurrence after a standard catheter ablation procedure, showing favourable results.51
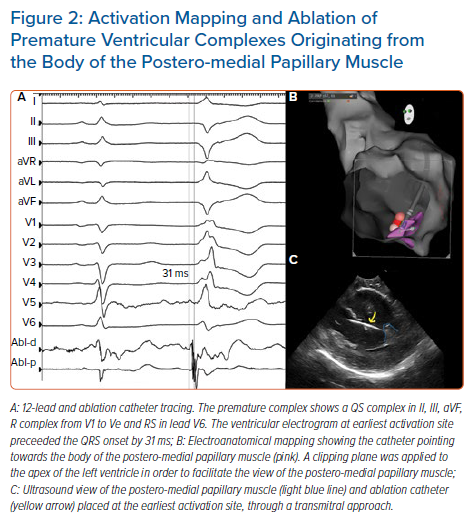
In a small cohort of patients, radiofrequency ablation with an 8 mm catheter tip was more effective than non-irrigated 4 mm tip catheter ablation.52 However, such a large bipolar dipole is not able to correctly identify low-voltage signals; the use of small and closely spaced diagnostic electrodes on a large-tip ablation catheter might allow both the detection of low-voltage fragmented electrograms and the application of deep lesions.53 Initial reports on a circumferential ablation around the base of the PM suggested a higher success rate.54 However, we believe that this strategy requires the delivery of a significant amount of radiofrequency, which could potentially damage PM architecture and, finally, increase the risk of complications.
Conclusion
MVP is now recognised as a cause of VAs and SCD that is not to be overlooked. The increasing interest in the pathophysiology and risk stratification of MVP-related arrhythmias allows us to more precisely target the arrhythmic substrate and to individualise therapies such as ICDs and catheter ablation. The recent technological advances in the field of catheter ablation need more extensive validation in MVP patients. Furthermore, randomised controlled clinical trials are welcomed to better understand indication, timing and outcomes of antiarrhythmic therapies in this complex but fascinating setting.
Clinical Perspective
- Specific subsets of patients with mitral valve prolapse have a significant risk of ventricular arrhythmias.
- Myocardial fibrosis and premature ventricular complexes may act as a substrate and trigger of malignant ventricular arrhythmias.
- Catheter ablation of ventricular arrhythmias in this setting is a promising therapeutic option; potential beneficial effects should be validated.