The cornerstone of catheter ablation for AF was the discovery in the late 1990s of rapidly firing ectopic foci from the pulmonary veins (PV) initiating AF, and the observation that ablation of these ectopic foci could abolish the arrhythmia.1 Additional recognition of the complex tissue architecture of the PV-left atrial (LA) junction led to a progression in catheter ablation approaches from focal PV ablation to ostial segmental ablation and subsequently to wide area circumferential ablation incorporating part of the posterior LA wall and the complex PV-LA junction.2–4 Randomised controlled trials and meta-analyses have since demonstrated increased effectiveness of this antral approach, which is also thought to result in lower risk of pulmonary vein stenosis.3,5,6 In patients with paroxysmal AF, outcomes are good. At 12 months, success rates approach 65%, increasing to 80% following repeat procedures.7–11 However, the observation of late recurrences with long-term follow-up despite pulmonary vein isolation (PVI) and the significantly lower success rates in patients with persistent arrhythmia highlight the importance of additional non-pulmonary vein mechanisms in AF initiation and maintenance.7,8,12–14
Although several empirical ablation strategies including linear ablation of the LA roof and mitral isthmus or ablation of complex fractionated atrial electrograms have been employed, none have been shown demonstrably to result in incremental benefit beyond a strategy of PVI alone, which, in the STAR-AF 2 trial, resulted in a 59% rate of freedom from AF recurrence after 18 months of follow-up.15–19 Recent trials of PVI in combination with posterior wall isolation have been similarly disappointing.20,21 Improved outcomes have been identified in small trials of strategies employing extensive catheter and/or surgical ablation techniques targeting a large volume of atrial myocardium.22–24 However, the potential development of stiff atrial syndrome resulting from such extensive iatrogenic scar formation risks counteracting the benefits of sinus rhythm restoration.25
Limitations of empirical strategies have subsequently led to attempts to develop individualised, patient-specific approaches targeting either localised areas of fibrosis or electrophysiological mechanisms.26 Results from animal models have suggested the existence of high-frequency localised and repetitive re-entrant circuits termed rotors, thought to represent ‘drivers’ responsible for maintenance of AF propagation.27–30 Early efforts to develop methods able to identify these mechanisms and thus guide ablation strategies showed promising results.31–35 However, recent randomised studies have been disappointing.21,36,37 These methods have largely relied on either dominant frequency analysis aimed at identifying zones of high-frequency activation, or phase mapping applied either to multi-electrode basket catheters or non-invasive body surface electrodes, which aim to identify phase singularities representing the pivot point of localised rotors.38 Further studies and evaluation of these techniques have revealed contrasting results and highlighted the limitations of these approaches.39–44
Charge density mapping (Acutus Medical) uses a non-contact approach to facilitate whole chamber mapping of atrial activation with the aim of identifying patient-specific electrophysiological mechanisms responsible for AF maintenance that can be targeted with ablation. In this review we aim to provide an overview of the background to this technology, the current clinical application and available evidence, as well as provide insights into the potential future benefits this technology may hold for patients.
Charge Density Non-contact Approach
The AcQMap system (Acutus Medical) is a combined imaging and electrophysiological mapping system that uses a diagnostic catheter comprising six splines, each of which incorporates eight ultrasound transducers interspersed with eight biopotential electrodes (Figure 1A). Through processing of up to 115,000 ultrasound points/minute, a high-resolution 3D reconstruction of the atrial chamber anatomy is generated within a few minutes (Figures 1B–1E and Supplementary Material Video 1). This is based on a triangular mesh structure comprising constituent corners (termed vertices) of shared triangular faces, which is integrated into a registered coordinate system for spatial localisation based on tracking within an impedance field. The distance between vertices is approximately 1.5–2 mm, which represents the spatial resolution of the system. The 48 biopotential electrodes record raw intracardiac unipolar signals at a rate of 150,000 samples/second. An inverse solution is applied to reconstruct unipolar signals at each unique point (approximately 3,500 vertices) on the generated endocardial surface anatomy. The use of regularisation as well as the proximity of the electrodes to the potential source on the atrial endocardium serve to limit any errors introduced in the solution to the inverse problem.
Uniquely, this system uses charge density mapping to provide sharper, high-resolution visualisation of cardiac activation in comparison with voltage signals.45 Cardiac electrograms measured from either non-contact electrodes in the cardiac chamber, electrodes in contact with the cardiac tissue or from the body surface, are measures of electric potential in units of volts. However, this electric potential does not originate in isolation, but rather originates from the presence of charge. The summation of charge, in varying magnitudes and location, directly affects the structure and magnitude of the electric potential field, and thus the voltage measured with an electrode at a given location.
As an action potential is generated within the myocardium, facilitated by the movement of ions across the cell membrane, a small charge imbalance is created in the region of the adjacent extracellular space. Initial depolarisation is followed by recruitment of adjacent cells and spreads outwards, resulting in a macroscopic distribution of charge, a ‘density’ over space, with the largest gradient of charge density at the depolarising wavefront. This results in an ability to represent the cardiac signals in a more spatially compact and morphologically narrow waveform – the charge density electrogram.45
These local charges represent the sources of the potential field measured as an electrogram by conventional electrophysiological recording systems or as an electrocardiogram when measured on the body surface. The relationship between charge and potential (voltage signals) is fundamentally defined by Poisson’s equation, allowing direct computation of the potential field caused by a given electric charge. Although charge itself cannot be directly measured, a set of potential field measurements can be used to inversely solve Poisson’s equation to determine the originating charge distribution giving rise to them.46 Following subtraction of the far-field QRS-T wave signals, the AcQMap non-contact approach relies on this technique to calculate the continuous chamber-wide, distribution of charge that gives rise to any ongoing cardiac activity, critical to the visualisation of complex and temporally variable AF propagation.
Validation has been performed examining the reconstructed voltage signals against contact unipolar electrograms recorded by a multipolar circular mapping catheter during both sinus rhythm and AF.47 Validation measures assessed included both electrogram morphology and timing. During sinus rhythm, median morphology cross-correlation and timing difference was 0.85 (interquartile range [IQR] 0.71–0.94) and 6.4 ms (IQR 2.6–17.1), respectively, with values of 0.79 (IQR 0.69–0.88) and 14.4 ms (IQR 6.7–26.2), for morphology and timing difference during AF.47 The radial distance between the centre of the AcQMap catheter and the atrial surface significantly impacted results, particularly at distances ≥40 mm (Figure 2).47
Activation Pattern Characterisation
Annotation of the negative slope of the charge signal, which correlates with phase 0 of the action potential, identifies the zone of depolarisation at any instant in time and enables construction of a propagation history map without the need for a stable timing reference.45 This is visualised as a moving leading edge of a wavefront displayed as an isochronal map, with differing isochronal spacing representing changing conduction velocities. Visual interpretation of the propagation map is complemented by the integrated, automated AcQTrack platform, which tracks and analyses the spread of the activation wavefronts between all adjacent vertices on the chamber anatomy. While smooth planar wavefronts are discounted, directional changes in propagation are identified and characterised.
Three distinct patterns of propagation are identified including focal firing (FF), localised rotational activation (LRA) and localised irregular activation (LIA) (Figure 3 and Supplementary Material Videos 2, 3 & 4). FF is identified if the activation at a vertex occurs at least 3 ms prior to all its immediate neighbours and is seen to propagate outwards centrifugally. LRA is identified by recording the degrees of conduction around a central point by calculating the cumulative angle differences of sequential conduction velocity vector directions around the central point and within a discrete area of approximately 300 mm2. If the rotational angle of conduction exceeds 270°, equivalent to a cumulative angle of wavefront activation change approaching 360°, rotation is detected at the central point. LIA is characterised by more complex directional changes in propagation but incorporates directional changes in activation differently from the criteria for LRA. Angle differences between the vector of a wavefront entering and leaving a confined region of approximately 200 mm2 are computed and if the difference exceeds 90°, LIA is detected.
Practical Considerations and Insights into AF Mechanisms
A major challenge in designing non-PV ablation strategies for persistent AF is the limitation in our understanding of AF mechanisms. Competing theories of AF propagation revolve around questions of hierarchical versus anarchical mechanisms and the role of structural versus functional substrate properties.48 Similarly, identification of appropriate ablation targets guided by charge density mapping is influenced by a combination of practical aspects of how the technology is implemented into a procedure and understanding of the mechanistic significance of the patterns of AF activation identified.
Ablation, by definition, delivers a fixed therapy. This is an important consideration in designing strategies aimed at AF activation patterns. Targeting a fixed therapy to transient or migratory phenomena is unlikely to be effective, while sites of highly repetitive and spatially stable activation patterns represent more attractive ablation targets. An appropriate duration of mapping that reveals these stable phenomena, together with an understanding of their spatial stability over time, is therefore required. We have previously demonstrated that regions of high-frequency LIA show a high degree of spatiotemporal stability, in contrast to regions of LRA that show the least consistency over repeated mapping segments.49 Although isolated focal activations are frequently observed throughout the chamber, zones of high-frequency FF (occurring at a frequency of ≥1 every 3 seconds) demonstrate greater stability. Similarly, while mapping durations of 12 seconds are adequate to identify these stable zones of LIA, 20–25 seconds are required to reveal repetitive zones of either LRA or FF.
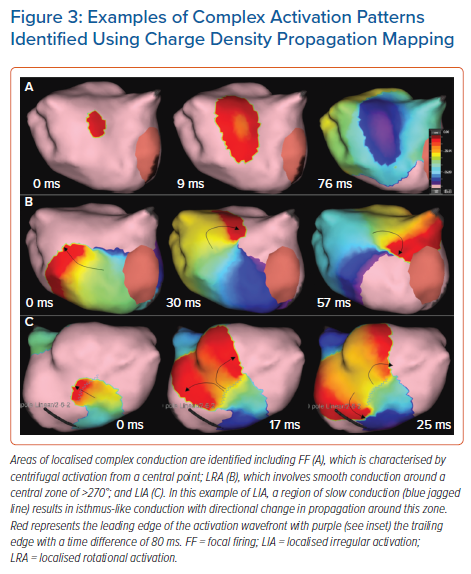
Lee et al. have recently published similar findings with high temporal stability of LIA and no meaningful relationship to low-voltage amplitude.50 Although bipolar voltage amplitude in regions of repetitive LIA appears preserved, these sites demonstrate an increase in conduction heterogeneity during short-coupled extra-stimulus pacing not observed in the remainder of the chamber.51,49 Considering the high degree of spatial stability, this suggests a relationship to underlying atrial structural properties with a potential role in AF maintenance, making sites of high-frequency LIA attractive targets for catheter ablation. From a practical perspective, analysis of a minimum of two to three mapping segments of 10 seconds duration is therefore required to accurately reveal the most stable phenomena and guide delivery of therapy.
In addition, there appears to be an inverse association between the frequency of focal and rotational activation patterns. Termination of AF during catheter ablation is associated with a higher frequency of FF, and patients with paroxysmal, compared to persistent AF demonstrate more FF with less LRA. Furthermore, we have shown that adenosine, which is known to shorten atrial refractoriness and provoke AF, promotes LRA alongside an acceleration in global AF cycle length. These findings suggest that LRA frequency may serve as a useful surrogate marker of atrial functional properties, but that transient and meandering LRA patterns represent unattractive targets for ablation.
The relative properties of LIA, LRA and FF may represent variation in their role in the maintenance of AF propagation and/or the progression from a trigger-dependent, to a substrate-dependent, trigger-independent mechanism. These differences may also reflect distinct phenotypes either reliant on focal ‘drivers’ to sustain downstream fibrillatory conduction, or able to self-sustain as a result of ‘maintainers’ that facilitate wave-break together with wavefront renewal processes perpetuating AF.52 These observations are important considerations in designing individualised ablation strategies and inform the hierarchy of ablation targets chosen. When present, high-frequency FF is targeted with the aim of eliminating AF ‘driver’ mechanisms, while more prevalent sites of LIA are ablated with a view to altering the substrate responsible for the maintenance of AF propagation.
These mechanisms described are distributed across both the LA and right atrium (RA). Much of the focus of work exploring AF mechanisms has been on the LA, without considering a bi-atrial perspective in AF maintenance. Simultaneous bi-atrial mapping using two linked AcQMap systems has helped to characterise the role of the RA. In most patients, AF propagation is maintained through balanced conduction between both chambers, while where one chamber dominates, this is as often the RA as the LA. In addition, electrophysiological substrate properties of the RA, including increasing frequency of LRA and shorter RA AFCL, but not the LA, appear to predict a failure of acute AF termination with ablation.
Ablation Strategy
Sites of repetitive LIA are most frequently distributed within the anterior and posterior LA and the septum and lateral walls of the RA, while zones of high-frequency FF are similarly distributed in addition to originating from the PVs.53 Importantly, these regions are commonly found in close proximity to the PV antra (particularly on the posterior wall), likely reflective of the complex structural properties of the PV-LA junction. Mapping prior to PVI allows incorporation of these sites within the wide antral lesion set. Repeat mapping both prior to and following PVI provides the additional benefit of revealing non-pulmonary vein sites with consistent patterns of activation across both timepoints that can help to determine the hierarchy of ablation targets.
We have described a ‘core-to-boundary’ approach that aims to deliver focused ablation to the core of sites of preferential conduction patterns while anchoring these regions to non-conducting boundaries to reduce the risk of generating substrate for organised macro-re-entrant atrial tachycardias (AT).54 This strategy compliments an approach of ‘map, ablate, re-map’ that takes advantage of the speed of map recording and generation to allow repeated evaluation of the impact of ablation delivered, alterations to the dynamic patterns of AF activation and identification of stable or emerging areas of interest.
The number of conduction pattern sites appears related to the individual AF phenotype and is greater in patients in AF at the start of the procedure, compared to patients in whom AF is induced, and is proportional to the duration of persistent AF.54,55 Although definitive evidence of the optimal endpoint for ablation is lacking, we propose a strategy focused on sequential ablation of sites of preferential conduction patterns across both chambers until either all sites are eliminated or AF terminates to either AT or sinus rhythm. This supports a low threshold for mapping and ablation of RA mechanisms following ablation in the LA but without incremental addition of empirical ablation with the aim of achieving sinus rhythm in all patients. In an unselected group of 135 patients undergoing AcQMap guided de novo or re-do ablation of persistent AF in our centre, acute termination with ablation is seen in 46%, reducing to 28% in patients attending the procedure in AF (as opposed to those in whom AF was induced). Evidence of correlation between acute AF termination and long-term procedural success is weak and therefore this should not be considered a definitive endpoint to be achieved in all patients after the elimination of targeted mechanisms.56
It should also be remembered that approximately 60% of patients undergoing first time procedures for persistent AF achieve good results from PVI alone.19 Where adoption of an individualised approach may prove particularly beneficial is in identifying those patients likely to do well with a strategy of PVI alone and limiting additional non-PV ablation only to those patients in whom this is likely to be required. As described above, the relative balance of FF and LRA appears related to acute AF termination. Different phenotypes can also be discerned in the pattern of whole chamber activation, with some patients demonstrating more ‘organised’ whole-chamber activation while others appear more chaotic and disorganised. Further work is needed to correlate these findings with long-term outcomes, but adapting the strategy to minimise additional ablation in patients with more organised whole chamber activation, while delivering more extensive ablation to bi-atrial AF mechanisms in others may prove most beneficial.
Mapping of Organised Atrial Tachycardias
ATs may arise either de novo or during AcQMap guided ablation of AF. The development of an algorithm for high-density mapping of stable atrial rhythms including both AT and paced rhythms provides more comprehensive utility of the AcQMap system beyond its application to mapping of AF and obviates the need for a second parallel mapping system. This algorithm, termed SuperMap accumulates multiple non-contact measurements acquired at different times and at different locations of the chamber by aligning beats according to timing and morphology of simultaneously recorded unipolar electrograms within the coronary sinus (CS). This allows separate grouping of beats with different cycle lengths or CS electrogram morphologies (for example, in the case of non-sustained tachycardias or unstable cycle lengths) that can be mapped concurrently and analysed separately. The atrial surface in proximity to the roving electrodes is highlighted during data collection to guide adequate spatial distribution of measurements. Charge densities are then computed by grouped beats, and the propagation history, an animated leading wavefront with color-coded activation histories, is generated for visualisation (Supplementary Material Video 5).
Ramak et al. have published the first description of the utility of this algorithm in a small series (n=7) of patients undergoing ablation of complex ATs following AF ablation.57 Shi et al. applied computational modelling to identify the optimum minimum number of catheter positions required to achieve a threshold of accuracy in local activation time annotation. A total of 60 separate locations was shown to meet this accuracy threshold which was achieved within a maximum of 3 minutes of continuous catheter roving. This formed the basis for subsequent clinical evaluation in a cohort of 20 patients undergoing ablation of ATs. Non-contact electrogram morphology and timing correlated well with contact electrograms obtained using the HD-grid (Abbot Medical).58
We have performed a systematic evaluation in 30 patients and a total of 60 separate maps including a comparison to contemporary contact mapping in 20 of these patients (42 individual maps). The SuperMap algorithm performed favourably in comparison to high-density contact mapping, accurately revealing the arrhythmia mechanism in 93% of arrhythmias evaluated. This was achieved over a 37% shorter procedure time, requiring an average of 374 ± 214 seconds (6 minutes, 14 seconds) compared to 591 ± 332 seconds (9 minutes, 51 seconds) using contact mapping (p<0.0005). This was achieved alongside a high number of electrograms used for each map (7,201 ± 5,276 versus 3,380 ± 3,234; difference 3,821; 95% CI [2,207–5,435]; p<0.0005), therefore not compromising on mapping density.59
Gold-tip Ablation
The non-contact diagnostic capability of the AcQMap system is complemented by radiofrequency (RF) ablation therapy based around a 3.5 mm gold-tip contact-force sensing ablation catheter (Acutus Medical in partnership with Biotronik), with impedance-based catheter localisation. Gold is well known to exhibit a significantly higher thermal conductivity compared to platinum, which represents the most widely used material for catheter tip electrodes. Delivery of RF energy during ablation is limited in part by rising temperature at the catheter–tissue interface that risks excessive heating of the blood pool and formation of coagulum with associated risks of embolism.
The superior thermal conduction properties of gold have been found to result in deeper and broader ablation lesions when compared to platinum electrodes in vitro using both 4- and 8-mm electrode sizes.60,61 Although this difference is mitigated in part by use of irrigated tip catheters, in a randomised study of cavo-tricuspid isthmus ablation the use of gold-tip catheters resulted in a higher procedural success rate.62 In addition, gold allowed delivery of a higher mean power alongside lower catheter tip temperatures that resulted in a significantly lower rate of coagulum formation.62 Similar findings have been observed during irrigated RF ablation of the pulmonary veins with lower tip temperatures and higher energy delivery.63 These properties also allow RF delivery at significantly lower irrigation flow rates, with potential benefits particularly in patients with impaired left ventricular function, who form a significant proportion of patients now undergoing catheter ablation for AF.63
Contact force capability is integrated into the catheter thereby making this the only contact force sensing gold-tip ablation catheter commercially available, which can be used either in conjunction with the AcQMap mapping system or as a standalone ablation system. Lesion formation is informed by measurement of force-time integral during RF delivery. Contact force is measured using Fiber Bragg Gating (FBG) technology, which detects changes in the reflected wavelength of a light source that occur due to mechanical distortion (force). Changes in the reflected wavelength can also be caused by temperature changes thereby impacting on the accuracy of contact force measurements obtained.
Uniquely, the AcQBlate catheter incorporates an additional FBG system within a non-deformable section of the catheter in which measurements obtained reflect temperature changes and are used to provide constant correction to the contact force measurement obtained from the tip sensor. Acutus Medical commercial testing (unpublished data) when compared to the TactiCath SE (Abbott Medical) contact force ablation catheter suggests improved accuracy of contact force measurement both during and after RF ablation delivery (Figure 4). In this analysis conducted in vitro, a constant reference force of 1 g was applied and compared to the measured force detected by AcQBlate and TactiCath SE catheters during and immediately after RF ablation delivery. Wider variation was observed in force measured using TactiCath, which remained at 4 g after cessation of RF while the value returned to 1 g applied force after RF termination with the AcQBlate system (Figure 4).
Clinical Outcome Data
The UNCOVER-AF trial was the first outcome study performed using the AcQMap system and was a single arm prospective study including 129 patients who underwent first time ablation procedures for persistent AF across 13 centres in the UK, Europe and Canada.55 Patients included were aged 62.4 ± 8.6 years with 3.2 ± 3.9 years since AF diagnosis, including 1.9 ± 3.1 years since onset of persistent AF. The mean LA diameter was 43 ± 4 mm and 46% of patients had a CHA2DS2-VASc score of ≥2. Outcome assessment included both safety and effectiveness, with arrhythmia recurrence detected using a combination of 12-lead ECG and 24-hour continuous ECG monitoring at 3, 6, 9 and 12 months following the procedure.
Major adverse events within 24 hours of the procedure were observed in three patients and consisted of cardiac tamponade (n=2) and cerebral thromboembolism (n=1). In addition, one patient suffered an air embolism related to the steerable sheath within the LA and two patients suffered complications related to the femoral venous puncture site. Ablation resulted in termination of AF in 32% of patients with 68% undergoing direct current cardioversion (DCCV) and two patients remaining in AF at the end of the procedure. Of those in whom ablation resulted in AF termination, an average of 2.4 ± 1.5 non-PV sites were targeted, which increased to 3.4 ± 1.4 in those in whom DCCV restored sinus rhythm. The primary outcome of freedom from AF >30 seconds in duration at 12 months (on or off antiarrhythmic drugs) was achieved in 72.5% of patients, with a freedom from AF or AT in 69.2%. Although this compares favourably to results from STAR-AF2, the more recent VENUS randomised controlled trial, and the PRECEPT study, direct comparisons to results from alternative ablation strategies cannot accurately be drawn without a control arm and randomisation.19,24,64
We have recently published 2-year outcome data from a series of 40 patients treated across two UK tertiary centres in which we described our ‘core-to-boundary’ approach to the first-time ablation of persistent AF. In addition, we conducted a propensity matched comparison against a strategy of PVI combined with posterior wall isolation (PWI), which has become a widely adopted empirical approach. Patients treated using the AcQMap system had a 68% rate of freedom from recurrent AF or AT off antiarrhythmic drugs after a single procedure, which compared favourably with the 46% rate of freedom from arrhythmia seen in the PVI and PWI group (p=0.043).54
Although these data show significant promise in terms of clinical success using an AcQMap-guided approach, further evidence is eagerly awaited. The RECOVER trial (NCT03368781) is a single-arm study mirroring the design of UNCOVER-AF in patients undergoing re-do ablation procedures for AF, which has completed recruitment and is at the last stages of follow up, while the DISCOVER study (AcQMap Registry; NCT03893331) is a registry study aimed at delivering real-world data regarding utility and clinical outcomes in a target population of 500 patients undergoing treatment using the AcQMap system. However, the data available to date and on the horizon are poor substitutes for well-conducted randomised controlled trials and definitive evidence of benefit for patients undergoing ablation for AF will not be possible without this data.
Future Perspectives and Gaps in Evidence
Although the AcQMap system contains the capability to collect contact mapping data, we have not discussed this as it is not part of the usual workflow in global substrate mapping and ablation of AF, although it is likely to be the subject of future technological development. We have demonstrated some of the insights that the AcQMap system has provided into mechanisms of AF and the potential benefit this may provide in terms of clinical outcomes.
There is much still to learn about the mechanisms of AF and how to optimally employ or interpret the mapping data generated. We have shown that regions of LIA are highly stable and likely to represent structural atrial properties, but it is unclear whether these sites are pathological, perhaps related to interstitial fibrosis or myofibre disarray, or are related to normal regions of changing fibre orientation and/or inter-atrial connections.
Detailed studies employing MRI and histological analysis are needed to explore this further. What is the optimal ablation approach? Should regions of FF be preferentially targeted, in keeping with the hypothesis of focal drivers, or should stable regions of LIA be treated as these are the regions of abnormal tissue responsible for fibrillatory conduction? What is the appropriate endpoint for ablation and how can the effect of ablation be accurately assessed and quantified in real-time to guide therapy? We have shown evidence highlighting the role of the RA but predicting which patients require RA ablation is difficult and not possible based on current mechanistic understanding.
In addition to furthering mechanistic understanding is the potential for technological developments that may yield further clinical application and advances. The ultrasound capability of the system is currently employed purely to generate endocardial surface anatomy. However, the ultrasound data generated can also be processed to yield data on atrial mechanical function, visualise contraction and potentially evaluate wall thickness and adjacent structures, which will greatly expand the overall system capability and application.
Use of the AcQMap catheter is also currently limited to the atria. A global mapping approach could be highly desirable within the ventricles both for mapping single or infrequent ectopy and for rapid assessment of ventricular tachycardia mechanisms that are haemodynamically poorly tolerated. Animal studies in this area are on-going and catheter adaptations to suit deployment within the ventricles are under investigation.
Conclusion
AcQMap is a non-contact charge density electrophysiological mapping system for the assessment of atrial arrhythmias that uses ultrasound reconstruction of atrial anatomy. This is coupled with a RF ablation delivery system based around a gold tip contact-force sensing ablation catheter. Visualisation of whole chamber activation and localised patterns of complex conduction during AF allows delivery of an individualised approach to catheter ablation incorporating non-pulmonary vein mechanisms of AF propagation.
A parallel algorithm for the synchronous non-contact mapping of ATs facilitates accurate and rapid high-density evaluation of complex AT mechanisms. Early evidence of clinical outcomes is promising but randomised clinical trials are needed to ultimately test the benefit of this approach to treating patients with AF.
Supplementary videos:
- Supplementary Material Video 1
- Supplementary Material Video 2
- Supplementary Material Video 3
- Supplementary Material Video 4
- Supplementary Material Video 5
Clinical Perspective
- AF is a highly complex arrhythmia and significant challenges remain in developing successful strategies for catheter ablation, particularly beyond pulmonary vein isolation. The development of whole chamber non-contact charge density mapping provides a novel and unique approach to treatment.
- Detailed studies of the nature of complex activation patterns during AF have revealed important spatiotemporal properties of localised conduction that are important for the development of targeted ablation strategies.
- Emerging evidence supports the clinical benefit of an individualised ablation approach targeting complex patterns of local activation with anchoring of these sites to adjacent non-conducting structures.
- Non-contact mapping of organised atrial arrhythmias is a highly accurate and efficient technique for identification and ablation of atrial tachycardia.