The role of catheter ablation (CA) in the management of ventricular tachycardia (VT) is becoming increasingly relevant, having repeatedly shown its superiority to medical therapy in reducing the arrhythmic burden, thus improving prognosis and quality of life in patients with structural heart disease presenting with VT.1–4 In such patients, recurrent VT and heart failure (HF) status are connected by a bidirectional link. Structural and functional changes related to advanced HF, such as progressive myocardial fibrosis, adrenergic hyperactivity, mechanical and electrical remodelling, and metabolic dysregulation, all contribute to the genesis and maintenance of VT, which may occur in up to 30 % of these patients.5
Moreover, indicators of advanced HF, such as very low ejection fraction and advanced New York Heart Association (NYHA) functional class, have been associated with an increased rate of periprocedural complications, VT recurrence and mortality in patients undergoing CA of VT.6–9 Ventricular arrhythmias (VAs) may worsen HF status, increasing mortality and hospitalisations.5,7 In this setting, pre-procedural risk stratification to identify high-risk patients can allow for preprocedural planning and optimisation of overall clinical status before the procedure, improving patient safety and post-procedural outcomes. We summarise the strategies currently available to predict the risk of AHD and the evidence supporting the use of HF optimisation tools during CA of VT.
Incidence and Predictors of Acute Haemodynamic Decompensation
Acute haemodynamic decompensation during CA of VT has been defined by our group as sustained hypotension (i.e. systolic blood pressure <90 mmHg), despite increasing doses of vasopressors, that required emergent placement of a mechanical haemodynamic support (MHS; i.e. an intra-aortic balloon pump or left ventricular assist devices) and/or procedure discontinuation. We reported an AHD prevalence of 11 % in a series of 193 consecutive patients with drug-refractory scar-related VT referred to our institution for CA. The occurrence of AHD during the procedure was associated with an almost sixfold increased risk of death after the procedure. Overall, 16 % of the patients died during a mean follow-up of 21 ± 7 months: 50 % in the AHD group versus 11 % in the group without AHD (p<0.001).
Upfront identification of these patients is pivotal, given the strong impact on mortality. Using logistic regression analysis, we identified eight predictors associated with AHD: age >60 years (OR 3.24; 95 % CI 1.07–9.82; p=0.037); diabetes (OR 2.81; 95 % CI 1.15–6.90; p=0.024); ischaemic cardiomyopathy (OR 6.26; 95 % CI 1.81–21.62; p=0.004); left ventricular ejection fraction (LVEF) <25 % (OR 3.00; 95 % CI 1.27–7.07; p=0.012); chronic obstructive pulmonary disease (OR 5.46; 95 % CI 2.24–13.33; p<0.001); presentation with VT storm (OR 5.12; 95 % CI 1.84–14.22; p=0.002); NYHA functional class III/IV (OR 6.11; 95 % CI 2.53–14.75; p<0.001); and use of general anaesthesia (OR 3.56; 95 % CI 1.50–8.44; p=0.004).6 With the exception of general anaesthesia, all of these variables are non-modifiable markers of HF severity.
On the basis of this analysis, the PAINESD risk score was developed by rounding the OR value to the next integer including the following variables: chronic obstructive pulmonary disease (5 points); age >60 years (3 points); ischaemic cardiomyopathy (6 points); NYHA functional class III or IV (6 points); LVEF <25 % (3 points); presentation with VT storm (5 points); and diabetes (3 points), with the maximum score being 31 points (Figure 1). The risk of periprocedural AHD increased across tertiles of risk score and was 1 % for first tertile (≤8 points), 6 % for second tertile (9–14 points) and 24 % for third tertile (≥15 points) (Figure 1). The use of this score may allow identification of patients at either very low (1 % for score ≤8 points) or high (24 % for score ≥15) risk of periprocedural AHD and might provide a simple tool to select patients who might benefit from prophylactic placement of MHS devices.
Our findings were subsequently confirmed in an independent cohort of 93 patients with structural heart disease undergoing VT ablation. Patients who required emergent prophylactic percutaneous left ventricular assistance device (pLVAD) insertion due to AHD during the procedure had significantly higher PAINESD scores compared with a control group of patients who did not undergo pLVAD insertion (mean PAINESD score of 17.8 ± 3.8 in the rescue pLVAD group versus 13.4 ± 5.4 in the non-pLVAD group; p=0.01). Patients in the rescue pLVAD group also showed a significantly higher 30-day mortality compared with the non-pLVAD group (58.3 % versus 3.5 %; p=0.001).10
Similar findings have been recently reported in a large retrospective series of 2,061 patients with structural heart disease undergoing VT ablation included in the International VT Ablation Center Collaborative Group, which incorporates procedural and outcome data of 12 international sites that specialise in VT management. Patients who died within 30 days of the procedure had significantly higher PAINESD scores (16 ± 7) compared with those who died later (14 ± 6; p=0.006) or those who survived throughout the all-study follow-up (9 ± 6; p<0.001), as well as a higher requirement for periprocedural MHS (25 % versus 5 %; p<0.001) and higher rates of acute procedural failure (Figure 2).7
Pre-procedural Heart Failure Status Optimisation and Intra-procedural Haemodynamic Monitoring
In patients presenting with recurrent VT in the setting of structural heart disease, there are several factors that may precipitate the overall haemodynamic performance. Some of these are modifiable and related to the procedure, such as the use of general anaesthesia or inotropes/vasopressors.11 Others are related to the acute clinical presentation, such as hypotension due to refractory VT/VF or cardiac stunning due to repeated ICD shocks.
Accurate pre-procedural risk stratification is essential to minimise the risk of peri-procedural complications. Every effort should be made to optimise the haemodynamic status before the procedure, especially in patients presenting with severely depressed ejection fraction. When signs of volume overload (peripheral oedema, elevated jugular venous pressure) are present, decongestion should be pursued with the use of intravenous loop-diuretic therapy or continuous slow-flow ultrafiltration (in cases of diuretic resistance). In patients with low-output HF or frank cardiogenic shock, inotropic or vasodilator therapy may be required for stabilisation and to allow recovery of end-organ function.12
Unfortunately, complete optimisation of HF status is not always possible due to the high burden and incessant nature of VAs. In these cases, the use of MHS can lead to an overall improvement of heart mechanics, ventricular wall stress, myocardial oxygen consumption and end-organ perfusion. Invasive haemodynamic monitoring with pulmonary arterial catheters and arterial lines can allow for objective monitoring and tailoring of therapy to achieve haemodynamic goals. These concepts may be extended to intraprocedural monitoring and, in addition to cerebral oximetry (to evaluate cerebral desaturation during the procedure), may allow detection of early signs of AHD such as sustained hypotension, increases in pulmonary capillary wedge pressure, oliguria and increasing serum lactate.13,14
Haemodynamic Mechanical Support
In high-risk patients and especially in those in whom an adequate pre-procedural HF optimisation is not possible due to the characteristics of the clinical VT, prophylactic placement of MHS devices should be considered to prevent periprocedural AHD and potentially reduce mortality.
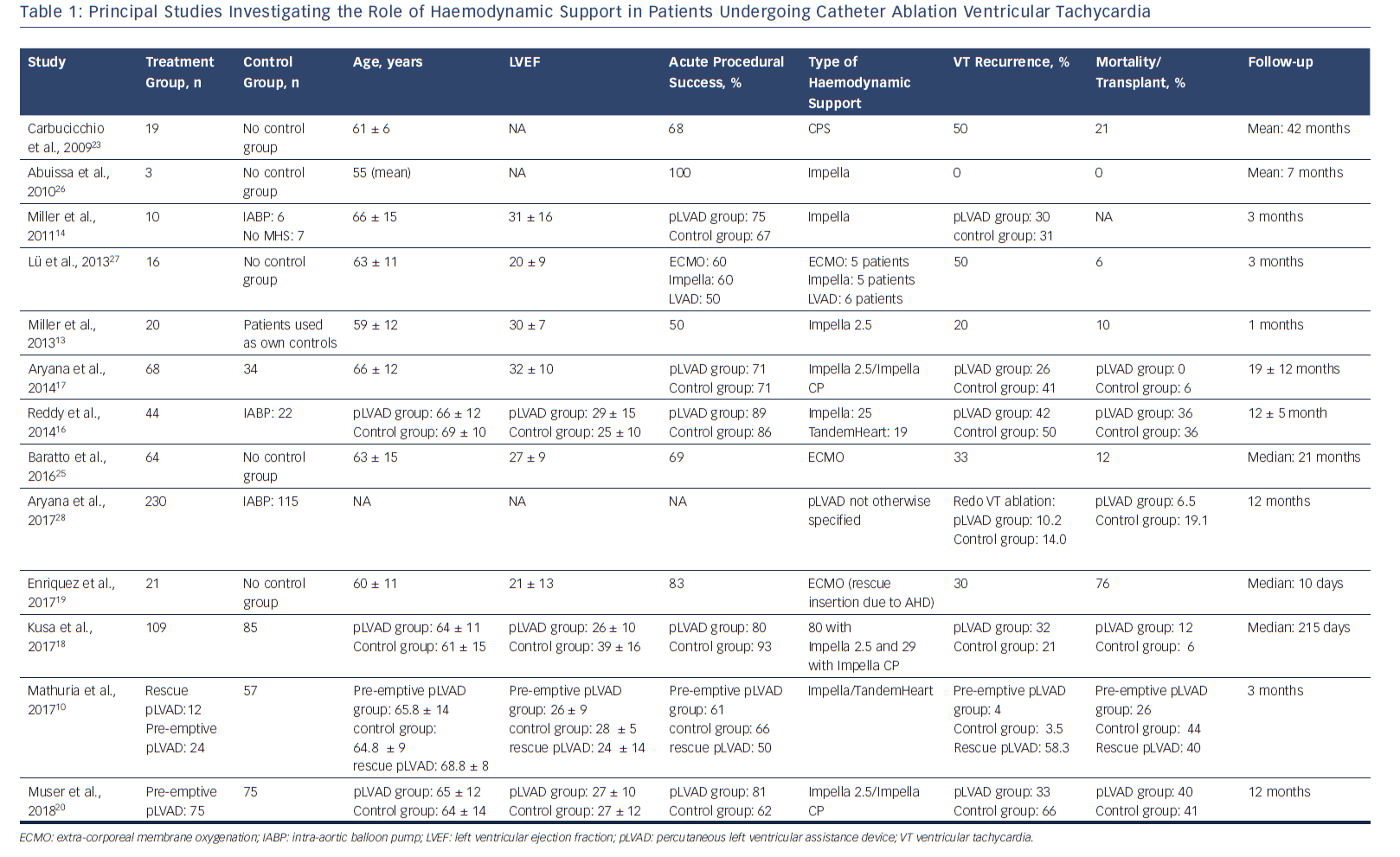

The main beneficial effects of MHS include maintenance of and adequate cardiac index and end-organ perfusion while promoting diuresis; reduction of intracardiac filling pressures, preventing significant increases in pulmonary pressures; reduction of left ventricular volume and wall stress; improvement of myocardial mechanics by reducing myocardial oxygen consumption; improvement of coronary perfusion; and support of systemic circulation and reduction of cardiac stunning due to multiple VT inductions for mapping and during ablation. However, the potential benefits of MHS must be weighed against the potential risks, such as arterial thromboembolism, bleeding and vascular complications at the insertion site.
Unfortunately, most of the available evidence supporting the use of haemodynamic support comes from retrospective registers or small observational studies with a substantial lack of specifically designed large randomised controlled trials (Table 1). Studies comparing the use of various types of MHS (i.e. Impella® [Abiomed Inc.], TandemHeart [CardiacAssist Inc.]) to the use of intra-aortic balloon pump (IABP) or no MHS at all have repeatedly demonstrated improvement in acute procedural outcomes by permitting mapping and ablation during sustained VT, improving the likelihood of achieving short-term VT termination.15 However, studies have not convincingly demonstrated a benefit on long-term VT-free survival.13,14,16–18
Even if the main goal of VT ablation is always elimination of VT, improving procedural safety and short-term post-procedural mortality are also important endpoints. Data regarding the impact of MHS on mortality are conflicting. In a recent study by Kusa et al. including 194 patients undergoing VT ablation (109 with the use of pLVAD and 85 without), no differences in the primary endpoint of recurrent VT, heart transplantation or all-cause death were seen between the two groups after a median follow-up of 215 days (primary endpoint: 36 % in the pLVAD group versus 26 % in the non-pLVAD group; p=0.14).18
A substantial lack of benefit related to the use of pLVAD was confirmed even after propensity score-matching analysis accounting for differences between the two groups, either in terms of acute procedural outcomes (VT inducibility at the end of the procedure: 14 % and 10 % in the p-LVAD and non-pLVAD groups; p=0.43) or long-term outcomes (death rate 5 % versus 8 %, p=0.50; heart transplantation rate 5 % versus 0 %, p=0.25; VT recurrence 26 % versus 21 %, p=0.29 in the pLVAD and non-pLVAD groups, respectively).Of note, in this series the decision for pLVAD insertion as well as its timing was made at the operator’s discretion considering haemodynamic instability during VT, frequency of VT episodes and severity of HF status. Therefore, both patients in whom the pLVAD was placed prophylactically and those in whom it was utilised as a ‘bailout’ therapy were included in the analysis, potentially affecting the outcomes.18
Mathuria et al. reported a higher 30-day mortality among high-risk patients undergoing rescue pLVAD placement after experiencing AHD during the CA procedure compared with high-risk patients in whom pLVAD was placed upfront (58.3 % versus 4 %; p=0.003). Interestingly, no significant difference in 30-day mortality was observed between high-risk patients in the prophylactic pLVAD group and low-risk patients in whom the procedure was safely terminated without the need for MHS (4 % versus 3.5 %; p=0.94) (Figure 2).10 These data suggest that the prophylactic (rather than rescue or bailout) use of MHS devices in high-risk patients undergoing VT ablation can result in the prevention of AHD and improved outcomes.
For example, our group has reported very poor outcomes in a series of 21 patients undergoing rescue cardiopulmonary support (CPS) with extra-corporeal membrane oxygenation (ECMO) for periprocedural AHD after CA of electrical storm. After a median follow-up of 10 days, 16 patients died while seven patients survived beyond 6 months post ablation, five remained free of VAs and three ultimately received a destination therapy (heart transplantation in two and LVAD in one). Among the patients who died, the cause of death was most commonly refractory HF (11 patients).19
We have recently confirmed these findings in a retrospective observational study comparing a group of 75 high-risk patients undergoing CA of scar-related VT in whom a prophylactic pLVAD (Impella) was implanted into a propensity score-matched control population of 75 patients who did not undergo prophylactic pLVAD placement.20 The PAINESD risk score was used for propensity matching. We reported the occurrence of AHD in 7 % of patients in the prophylactic pLVAD group and in 23 % of them in the control population (p<0.01). The 12-month cumulative incidence of VT was 40 % in the prophylactic pLVAD group versus 41 % in the control group (p=0.97), while the 12-month incidence of death/transplant was 33 % versus 66 %, respectively (p<0.01). At multivariable analysis, the use of prophylactic pLVAD was associated with a 3.5-fold reduction in the risk of death or transplant.20 Interestingly, by stratifying the patients according to their PAINESD score, patients at high risk (PAINESD ≥15) showed a substantial mortality benefit, with a 2.3-fold lower mortality risk among patients treated with pLVAD (HR 0.43; 95 % CI [0.21-0.87]; p=0.02). Meanwhile, in the low-risk patients (PAINESD ≤8; HR 0.63; 95 % CI 0.24–1.66; p=0.35) there was no statistically significant benefit of pLVAD, suggesting that the observed mortality benefit seen in the overall study group was largely driven by high-risk patients (Figure 2).20
An analysis of patients from the Medicare Inpatient Standard Analytic File database found that those who underwent VT ablation with pLVAD were less likely than those treated with IABP to develop periprocedural AHD (9.1 % versus 23.5 %; p<0.001) and acute renal failure (11.7 % versus 21.7 %; p=0.01), and had lower rates of death (6.5 % versus 19.1 %; p=0.001), 30-day all‐cause hospital readmissions (27.0 % versus 38.7 %; p=0.04) and HF‐related (21.4 % versus 33.3 %; p=0.03) hospital readmissions. This was despite the fact that those in the pLVAD group had higher incidences of HF than those in the IABP group (84.3 % versus 73.0 %; p=0.01). No significant difference was observed in terms of redo‐VT ablation rates at 1 year (10.2 % versus 14.0 %; p=0.34).17
The are several types of MHS currently available. The choice of a specific device is strictly dependent on patient characteristics like overall HF status and the presence of significant valvular disorders, such as moderate-to-severe aortic regurgitation and right ventricular dysfunction. IABP, TandemHeart left atrial-to-femoral artery bypass, Impella and ECMO have been described in CA of VT, and it is the largest published experience so far with the Impella and TandemHeart systems (Table 2).
IABP is frequently used in case of cardiogenic shock, especially in patients with severe coronary artery disease. The balloon is positioned in the descending aorta: it unloads during systole, decreasing cardiac afterload and inflates during diastole, augmenting diastolic pressure and therefore coronary artery perfusion with an overall improvement of the cardiac performance but without significantly impacting cardiac output (CO). Balloon counter-pulsation is triggered by pressure curve or ECG, limiting its performance in cases of fast/irregular rhythm.
The Impella MHS system is a continuous-flow axial pump placed through the aortic valve to pump blood directly from the left ventricle to the ascending aorta and able to provide a CO up to 5.0 l/min depending on the specific device used (Impella 2.5/CP/5.0). Use of Impella requires systemic anticoagulation with intravenous heparin to achieve an activated clotting time (ACT) >250 seconds to prevent stop of the system caused by thrombus formation. In cases of epicardial mapping/ablation, pericardial access should be obtained before Impella placement and absence of pericardial bleeding must be confirmed before starting anticoagulation. In cases in which pericardial access was not planned upfront, the device can be removed or withdrawn into the descending aorta, maintaining irrigation and a low performance level to avoid thrombus formation in order to reverse anticoagulation and safely gain pericardial access.21
The TandemHeart support system consists of a left atrial-to-femoral artery bypass able to provide up to 5 l/min of output by the use of an external centrifugal pump. Access to the left atrium is obtained by transseptal puncture; a bolus of heparin is given before transseptal puncture, followed by infusion to maintain an ACT >300 seconds.22 Some patients with advanced HF have significant biventricular dysfunction and LVAD support may be inadequate. In these cases, devices providing biventricular support such as ECMO should be considered.
Initial experiences with cardiopulmonary bypass used CPS, which consists of a centrifugal pump, an oxygen cylinder and a small heating system carried by a mobile cart and connected to the patient through an arterial and venous line. Percutaneous arterial cannulas typically measure 17–21 Fr, while venous cannulas range from 20–24 Fr. Adequate venous return is achieved by placement of the venous cannula inside the right atrium; the cannula is then connected to the inlet of the centrifugal pump, which pumps the blood through the oxygenator, heat exchanger and back to the patient.
The use of CPS has shown promising results: in a series of 19 patients undergoing VT ablation, only one patient needed an emergency heart transplant and eventually died during the index hospitalisation while three more patients died during a median follow-up of 42 months.23 However, CPS has several limitations: the combination of relatively small cannulas with pump-driven venous return allows flows between 3 and 4 l/min.
Moreover, the use of small-bore cannulas that may result in significant pressure gradients and eventually haemolysis, as well as the use of polypropylene oxygenators with porous fibres leading to plasma leakage and the use of non-thrombo-resistant surfaces makes CPS unsuitable for long-term applications.24 Most of these limitations were subsequently overcome by the use of ECMO that is designed for long-term (up to several weeks) circulatory support with flow >5 l/min. In particular, ECMO uses large-bore cannulas and specifically designed oxygenators either of true membrane type (continuous silicone surface precluding plasma leakage) or with relatively thrombo-resistant surfaces (heparin coated), allowing for less anticoagulation.24
In a recent study involving 64 high-risk patients undergoing CA of unstable VTs, pre-emptive ECMO was implanted in 59 patients, whereas rescue ECMO was used due to AHD in the remaining five patients. The use of ECMO allowed for the completion of the procedure in 92 % of the patients, achieving the endpoint of VT non-inducibility in 69 %, with an 88 % overall survival rate at 21-months follow-up. Only one patient did not survive to hospital discharge, one patient received LVAD, and three patients underwent heart transplantation.25
Conclusion
Periprocedural AHD is a serious complication that may occur in patients with structural heart disease undergoing CA of scar-related VT and is associated with an increased risk of mortality. The PAINESD risk score has demonstrated an ability to identify patients at risk of AHD and can be used as a clinical risk-stratification tool in patients undergoing CA of VT. In high-risk patients, prophylactic implantation of a MHS device should be considered to reduce the risk of AHD and post-procedural adverse outcomes.
Clinical Perspective
Acute haemodynamic decompensation (AHD) is a severe complication that may occur in up to 10 % of patients undergoing catheter ablation of scar-related ventricular tachycardia (VT) and is associated with increased post-procedural mortality. Clinical factors correlated with AHD include advanced age, ischaemic cardiomyopathy, severe heart failure status and presentation with VT storm, as well as comorbidities such as diabetes and chronic obstructive pulmonary disease. Those variables are included in the PAINESD risk score, which has been demonstrated as an accurate and reproducible tool to identify patients at high risk of AHD. Pre-procedural identification of such patients is pivotal in order to optimise heart failure status and to select the best candidates who may benefit from prophylactic implantation of mechanical haemodynamic support.